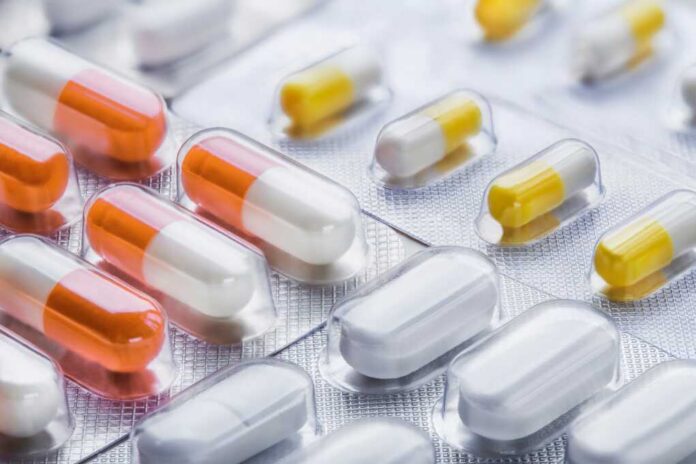
Scientists have uncovered how the FusB protein enables bacteria to resist fusidic acid by acting like a molecular crowbar to free trapped ribosomes, revealing nature’s sophisticated antibiotic defense mechanisms.
At a Glance
- Fusidic acid is a critical antibiotic against Staphylococcus aureus infections, including MRSA strains
- The antibiotic works by trapping elongation factor G (EF-G) on bacterial ribosomes, halting protein synthesis
- FusB protein functions as a “molecular crowbar” to dislodge fusidic acid from its target, restoring bacterial protein production
- Advanced imaging techniques have captured this resistance mechanism at the atomic level
- Understanding this process could lead to new strategies to overcome antibiotic resistance
The Battle Against Staphylococcus aureus
Staphylococcus aureus represents one of the most significant bacterial threats in both hospital and community settings, causing infections with high morbidity and mortality rates. Fusidic acid, derived from the fungus Fusidium coccineum, has emerged as a valuable weapon against these infections, particularly for methicillin-resistant S. aureus (MRSA). This antibiotic’s importance has grown as bacterial resistance to other antibiotics continues to rise. However, global surveillance data indicates concerning trends, with fusidic acid resistance rates reaching 0.5% for total S. aureus, 2.6% for MRSA, and a concerning 6.7% for methicillin-susceptible S. aureus (MSSA), with higher resistance rates particularly notable in European countries like Greece.
How Fusidic Acid Attacks Bacteria
The effectiveness of fusidic acid stems from its precise mechanism of action against bacterial protein synthesis. The antibiotic targets elongation factor G (EF-G), a crucial component in the protein production machinery of bacteria. During normal protein synthesis, EF-G helps move transfer RNA (tRNA) molecules through the ribosome in a process called translocation. Fusidic acid binds to the EF-G-ribosome complex, effectively locking it in place and preventing the vital dissociation of EF-G from the ribosome.
This binding action halts protein synthesis, ultimately leading to bacterial cell death. Importantly, fusidic acid doesn’t affect human cells due to fundamental differences in our protein synthesis machinery, making it a valuable clinical treatment.
The FusB Protein: A Molecular Crowbar
Recent research has revealed how bacteria fight back against fusidic acid through an ingenious resistance mechanism. The bacterial resistance protein FusB acts like a molecular crowbar, physically forcing the separation of fusidic acid from its target on the ribosome. Using advanced electron microscopy techniques, scientists have created what amounts to a “stop-motion movie” at the molecular level, capturing the precise action of FusB.
The protein strategically attaches to the bacterial ribosome where fusidic acid has trapped EF-G, applying mechanical force to break this interaction. This liberation allows the bacterial ribosome to resume its normal protein synthesis functions despite the presence of the antibiotic.
Multiple Resistance Strategies
Bacteria employ several different mechanisms to overcome fusidic acid’s effects. Beyond the FusB protein strategy, bacteria can develop mutations in the fusA gene that encodes the EF-G protein. These mutations alter the structure of EF-G, reducing fusidic acid’s binding affinity and effectiveness.
One notable mutation, F88L, causes slower tRNA translocation and ribosome recycling, initially creating fitness defects in the bacteria. However, bacteria can develop compensatory mutations, such as the M16I mutation, which work together with F88L to restore bacterial fitness while maintaining resistance. The position of Phe-88 in EF-G appears particularly crucial for fusidic acid binding and the functional conformational changes necessary for protein synthesis.
Plasmid-Mediated Resistance
Another sophisticated resistance strategy involves plasmid-mediated mechanisms. Bacteria carrying resistance genes on plasmids demonstrate a high basal level of resistance that can be rapidly induced to even higher levels after exposure to fusidic acid. This induction happens quickly and appears independent of gene dosage effects. Interestingly, in laboratory studies, protein synthesis in cell-free extracts from plasmid-mediated resistant staphylococci remains as sensitive to fusidic acid as in non-resistant organisms.
This suggests the resistance mechanism involves more complex cellular processes beyond simple target site modification. Some research indicates that expression of plasmid-mediated resistance correlates with changes in cell membrane composition, specifically alterations in phospholipid ratios.
Implications for Future Antibiotic Development
The atomic-scale understanding of how FusB dislodges fusidic acid from bacterial ribosomes represents a significant advancement in our knowledge of antibiotic resistance mechanisms. This detailed view of the molecular interactions involved provides crucial insights that could guide the development of new antibiotics capable of overcoming such resistance strategies. Future drugs might target multiple sites simultaneously or incorporate features that prevent the action of resistance proteins like FusB.
Additionally, combination therapies could be designed to inhibit these resistance mechanisms while delivering effective antibiotic treatment. As bacterial antibiotic resistance continues to pose a global health threat, these molecular insights offer valuable direction for researchers working to maintain our ability to treat dangerous bacterial infections.